Written by: Sarah Bowers, PhD Student, Brown Lab (right)
Edited by: Dr. Michael Hughes, Research Associate, McNagny Lab (left)

Figure 1. Modulating fibrinogen expression using lipid nanoparticle (LNP) delivery of small interfering RNA (siRNA) targeting the fibrinogen alpha chain (siFga). LNP-siRNA reagents (far left) administered intravenously (I.V.) reduce liver Fga mRNA levels (middle) and fibrinogen levels in platelets and plasma (far right) in mice. Adapted from: Juang et al. (2022)5.
Fibrinogen plays multi-faceted roles in many processes that are essential for the maintenance of human health and the prevention of disease1. The formation of a stable blood clot, for example, relies on thrombin-mediated cleavage of fibrinogen to fibrin1. On the flip side, elevated fibrinogen during inflammation (hyperfibrinogenemia) increases the risk of thrombosis2. Whereas extravascular fibrin(ogen) deposits at sites of tissue damage contribute to leukocyte recruitment and tissue repair3, the immunomodulatory activity of fibrin(ogen) may increase the metastatic potential of circulating tumour cells4. As such, strategies to fine-tune plasma concentrations of fibrinogen safely and effectively could have therapeutic utility1,5.
In a recent study published by the Kastrup Lab at the UBC Centre for Blood Research and Michael Smith Laboratories5, the authors investigated a strategy to modulate fibrinogen expression using lipid nanoparticle (LNP) delivery of small interfering RNA (siRNA) targeting the fibrinogen alpha chain (siFga) (Figure 1). Lead author Juang and colleagues describe how dose- and time-dependent control of fibrinogen levels can suppress fibrin(ogen)-driven pathologies in various disease models.
Although strategies for decreasing circulating fibrinogen have been investigated, strategies to safely and durably decrease fibrinogen concentrations do not exist. In the present study, administration of LNP-siFga to mice reduced liver Fga mRNA levels and fibrinogen levels in platelets and plasma. A dose-dependent decrease of fibrinogen in plasma was observed, with slowest recovery of fibrinogen levels at the highest dose. All levels returned to baseline after four weeks enabling the study of a range of fibrinogen levels over time. Although the majority of the work in this study involved controlled knockdown of mouse Fga mRNA, human-specific LNP-siFga also successfully supressed FGA mRNA in a human liver cell line.
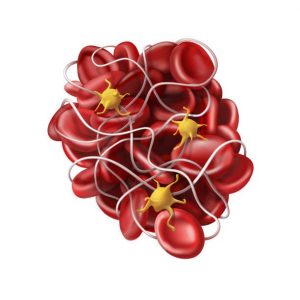
A cartoon blood clot, with red blood cells shown bound by white fibrin filaments. Fibrin formation involves thrombin-mediated proteolytic cleavage of fibrinogen. Credit: Getty Images/iStockphoto.
Furthermore, the authors investigated the effects of LNP-siFga administration on hemostasis using thromboelastography (TEG) to measure the stiffness of blood clots and mouse models of bleeding. Consistent with impaired hemostasis, the rate of clot formation and clot strength were significantly decreased in blood from siFga-treated mice. However, in mouse bleeding models, bleeding time and blood loss did not differ between siFga-treated or control mice, suggesting the impairment of hemostasis came with minimal bleeding risk.
Juang and colleagues then used three mouse models of disease to investigate the impact of LNP-siFga on fibrin(ogen)-driven pathologies. These included an endotoxemia (lipopolysaccharides-induced), peritonitis (thioglycollate-induced), and experimental lung cancer metastasis model. Beneficial outcomes of LNP-siFga administration were observed for all three models. In the endotoxemia model, for example, siFga administration attenuated hyperfibrinogenemia and markers of the acute phase response (a prelude to sepsis). Notably, in the lung cancer metastasis model, LNP-siFga treatment inhibited the metastatic potential of tumor cells to an extent comparable to that observed in fibrinogen-deficient mice.
Overall, the results of this study demonstrate the utility of LNP-encapsulated siFga as an experimental tool to investigate the role of fibrin(ogen) in a variety of fibrinogen-dependent processes. This method is considered a promising therapeutic strategy to manage fibrin(ogen)-dependent pathologies, although further work is needed to establish its clinical utility. To hear more from members of the Kastrup Lab about using siRNA gene therapy to understand the biochemistry of blood coagulation, check out this previous CBR blog post.
An article related to the Kastrup Lab’s study has been published in the journal Blood: “When fibrin(ogen) is too loud, silence it!”
References
1 Vilar, R., Fish, R. J., Casini, A., & Neerman-Arbez, M. (2020). Fibrin(ogen) in human disease: both friend and foe. Haematologica, 105(2), 284–296.
2 Machlus, K. R., Cardenas, J. C., Church, F. C., & Wolberg, A. S. (2011). Causal relationship between hyperfibrinogenemia, thrombosis, and resistance to thrombolysis in mice. Blood, 117(18), 4953–4963.
3 Luyendyk, J. P., Schoenecker, J. G., & Flick, M. J. (2019). The multifaceted role of fibrinogen in tissue injury and inflammation. Blood, 133(6), 511–520.
4 Palumbo J. S., et. al. (2000). Fibrinogen is an important determinant of the metastatic potential of circulating tumor cells. Blood. 96(10), 3302-3309.
5 Juang, L. J., et al. (2022). Suppression of fibrin(ogen)-driven pathologies in disease models through controlled knockdown by lipid nanoparticle delivery of siRNA. Blood, 139(9), 1302–1311.