Featuring: Hydrophilic Polymer-Guided Polycatecholamine Assembly and Surface Deposition
Yan Mei,† Kai Yu,† Hossein Yazdani-Ahmadabadi, Dirk Lange, and Jayachandran N. Kizhakkedathu*
ACS Applied Materials & Interfaces, 2022, 14, 39577-39590
Written by: Erik Lamoureux, PhD Student, Ma Lab
Edited by: Sarah Bowers, PhD Candidate, Brown Lab
In this piece, Erik Lamoureux (PhD Candidate, Ma Lab) introduces the unique challenges associated with biomaterial surface modifications in a biomedical setting, and a recent publication on studies by the Kizhakkedathu group at the UBC Centre for Blood Research (CBR) that could help address these challenges.
The modification of surfaces to produce innovative materials has allowed for the development of cutting-edge technologies ranging from automobile parts to electronics, and even biomaterials with applications in medicine.1–6
In the setting of organ transplant and surgery, there is a need for sutures and biomedical devices made of materials that function under wet conditions and on soft tissues, that are resistant to bacterial infection, and are biocompatible to avoid unwanted host immune responses. Although recent research has led to innovations such as self-dissolving (biodegradable) stitching materials, there remains a need for affordable and safe alternatives. Existing biomaterials do not provide adequate protection from risks such as implant-associated infection, where antibiotic-resistant biofilms may grow on the device, necessitating their removal. Additionally, foreign blood-contacting materials can trigger clot formation around the implanted device and/or in the circulation, resulting in serious and even fatal vascular events.
The development of coatings to modify surface properties for improved biocompatibility and hemocompatibility is a promising and important way for researchers to address these challenges.
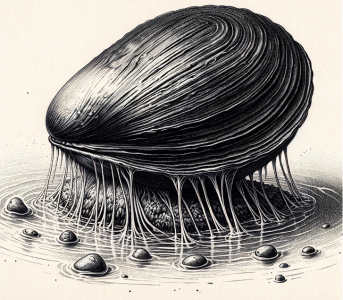
Figure 1. Stylized image of mussel adhering to a wet surface. The external “byssal” threads have remarkable chemical and material properties for attaching to wet surfaces, desirable for a range of biomaterials with clinical utility. Created with GPT-4’s DALL-E.
To inform their studies, scientists turned to sea mussels because these animals secrete a complex protein mixture that allows them to adhere to nearly all types of materials under dry and wet conditions (Figure 1). In fact, the chemical composition of an adhesive mussel protein informed the development of polydopamine (PDA) universal coatings which, along with other catecholamine-based coatings, are now used to modify material surfaces for biomedical applications.1,7–10
In a recent publication in ACS Applied Materials & Interfaces,1 the Kizhakkedathu group from the CBR studied the properties of (poly)catecholamines (e.g., polydopamine, PDA, and polynorepinephrine, PNE) with functional molecules that bind to the coatings.1,13–19 Using a one-step dipping process, nanoaggregates clump together to form nanoparticles large enough to form solids in solution and ultimately a crosslinked hydrophilic surface coating with anti-biofilm properties (Figure 2). Importantly, this co-deposition method has improved versatility and scalability compared to conventional (two-step dipping) processes.
The Kizhakkedathu group investigated co-deposition of (poly)catecholamines (e.g., PDA and PNE) and six different hydrophilic polymers noted for their anti-biofilm characteristics. The authors first evaluated the stabilization of the nanoaggregates and found that if interactions between PDA and its co-deposited polymer were too strong in solution, no nanoparticles were produced and the surface covering was not sufficient to prevent biofilm growth (e.g., PDA-PVP in Figure 2). When interactions between the PDA
and polymer were less strong, the cross-linked surface coating more effectively prevented biofilm growth (e.g., see PDA-PDMA in
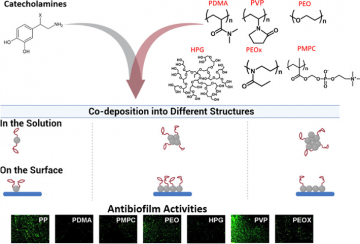
Figure 2. Catecholamines (e.g., PDA) and different hydrophilic polymers (e.g., PDMA) were co-deposited to produce anti-adhesive coatings on various substrates.1 At the bottom, fluorescent microscopy images in green representing bacterial growth, reveal anti-biofilm properties of the co-deposited solutions.
Figure 2). Coatings formed by the polymers were hydrophilic (water-loving), as indicated by water being spread out on the surface instead of “beading” as you would see on a water-proof raincoat. Desirable coatings not only need to be water-loving to integrate with the wet conditions of the body but also must not accumulate bacteria and other unwanted organisms. The researchers also evaluated the coatings’ anti-adhesive and anti-fouling properties, including low surface charge.20
Overall, the PDA-PDMA coating performed the best in resisting biofilm formation for two types of bacteria (Figure 2). Incredibly, co-deposition of PDA with PDMA afforded a 43-fold reduction in biofilm formation, as compared with a 6.7-fold reduction when the PDA coating was modified with PDMA post-deposition (the conventional two-step dipping process). Due to its versatility, simplicity, and scalability, dopamine-assisted co-deposition is a remarkable tool for developing novel biomaterials.
Overall, this work by the Kizhakkedathu group underlines the enormous value of co-depositing catecholamines with various polymers to improve the quality of antifouling biofilm-inhibiting coatings. The findings, which continue to rapidly evolve, is of major interest in the development of materials with enhanced biocompatibility, hemocompatibility, and resistance against bacterial infection.
Erik would like to thank Michael W. Kulka, Postdoctoral Research Fellow, formerly with the Kizhakkedathu group, for his helpful feedback on an early version of this article.
References
- Y. Mei, K. Yu, H. Yazdani-Ahmadabadi, D. Lange and J. N. Kizhakkedathu, ACS Appl. Mater. Interfaces, 2022, 14, 39577–39590.
- J. J. Richardson, M. Björnmalm and F. Caruso, Science, 2015, 348, aaa2491.
- Q. Wei and R. Haag, Mater. Horiz., 2015, 2, 567–577.
- Md. A. Rahim, S. L. Kristufek, S. Pan, J. J. Richardson and F. Caruso, Angew. Chem. Int. Ed., 2019, 58, 1904–1927.
- H. Ejima, J. J. Richardson, K. Liang, J. P. Best, M. P. van Koeverden, G. K. Such, J. Cui and F. Caruso, Science, 2013, 341, 154–157.
- Q.-Z. Zhong, S. Li, J. Chen, K. Xie, S. Pan, J. J. Richardson and F. Caruso, Angewandte Chemie International Edition, 2019, 58, 12563–12568.
- S. Hong, J. Kim, Y. S. Na, J. Park, S. Kim, K. Singha, G.-I. Im, D.-K. Han, W. J. Kim and H. Lee, Angewandte Chemie International Edition, 2013, 52, 9187–9191.
- P. Delparastan, K. G. Malollari, H. Lee and P. B. Messersmith, Angewandte Chemie International Edition, 2019, 58, 1077–1082.
- C. Lim, J. Huang, S. Kim, H. Lee, H. Zeng and D. S. Hwang, Angewandte Chemie International Edition, 2016, 55, 3342–3346.
- S. M. Kang, J. Rho, I. S. Choi, P. B. Messersmith and H. Lee, J. Am. Chem. Soc., 2009, 131, 13224–13225.
- W.-Z. Qiu, H.-C. Yang and Z.-K. Xu, Advances in Colloid and Interface Science, 2018, 256, 111–125.
- H.-C. Yang, K.-J. Liao, H. Huang, Q.-Y. Wu, L.-S. Wan and Z.-K. Xu, J. Mater. Chem. A, 2014, 2, 10225–10230.
- T. N. Gevrek, K. Yu, J. N. Kizhakkedathu and A. Sanyal, ACS Appl. Polym. Mater., 2019, 1, 1308–1316.
- H. Y. Ahmadabadi, K. Yu and J. N. Kizhakkedathu, Colloids and Surfaces B: Biointerfaces, 2020, 193, 111116.
- K. Yu, A. Alzahrani, S. Khoddami, D. Ferreira, K. B. Scotland, J. T. J. Cheng, H. Yazdani-Ahmadabadi, Y. Mei, A. Gill, L. E. Takeuchi, E. Yeung, D. Grecov, R. E. W. Hancock, B. H. Chew, D. Lange and J. N. Kizhakkedathu, Advanced Healthcare Materials, 2021, 10, 2001573.
- K. Yu, A. Alzahrani, S. Khoddami, J. T. J. Cheng, Y. Mei, A. Gill, H. D. Luo, E. F. Haney, K. Hilpert, R. E. W. Hancock, D. Lange and J. N. Kizhakkedathu, ACS Appl. Mater. Interfaces, 2021, 13, 36784–36799.
- H. Yazdani-Ahmadabadi, D. F. Felix, K. Yu, H. H. Yeh, H. D. Luo, S. Khoddami, L. E. Takeuchi, A. Alzahrani, S. Abbina, Y. Mei, L. Fazli, D. Grecov, D. Lange and J. N. Kizhakkedathu, ACS Cent. Sci., 2022, 8, 546–561.
- K. Yu, R. Warsaba, H. Yazdani-Ahmadabadi, D. Lange, E. Jan and J. N. Kizhakkedathu, ACS Biomater. Sci. Eng., 2023, 9, 329–339.
- Y. Mei, K. Yu, J. C. Y. Lo, L. E. Takeuchi, N. Hadjesfandiari, H. Yazdani-Ahmadabadi, D. E. Brooks, D. Lange and J. N. Kizhakkedathu, ACS Nano, 2018, 12, 11881–11891.
- S. Guo, D. Jańczewski, X. Zhu, R. Quintana, T. He and K. G. Neoh, Journal of Colloid and Interface Science, 2015, 452, 43–53.